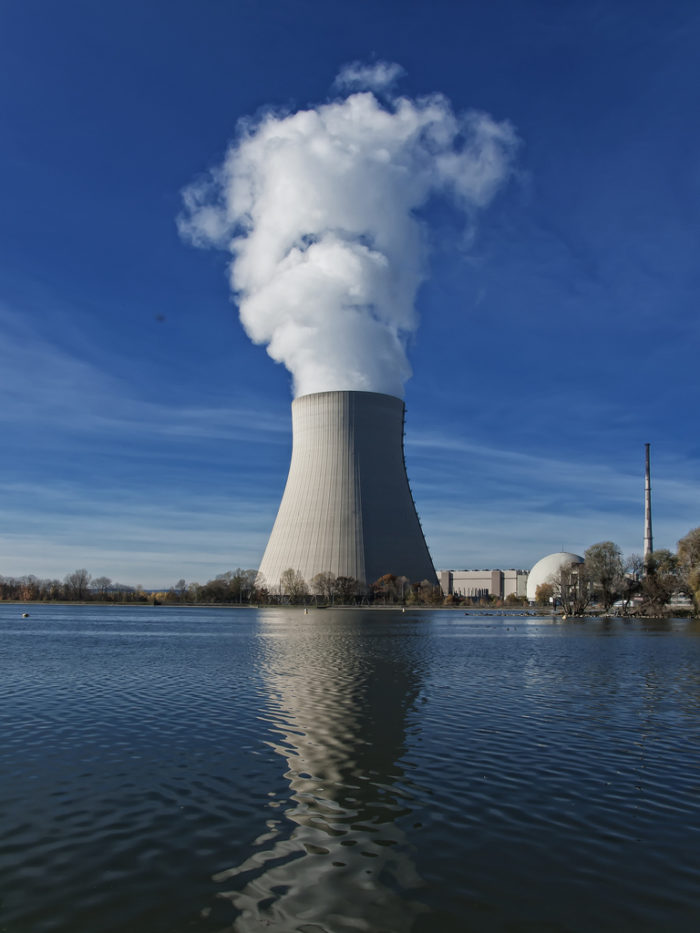
Image Credit: Bjoern Schwarz / CC BY 2.0K / Flickr
Nuclear power is dead. Long live nuclear power. Nuclear power is the only way forward. Nuclear power is a red herring. Nuclear power is too dangerous. Nuclear power is the safest power source around. Nuclear is nothing. Nuclear is everything.
It is now generally agreed that the world must rapidly reduce carbon emissions in order to fight off dangerous climate change, but the “how” of that process remains up for debate. And within that debate, nothing seems to produce such starkly opposing viewpoints as nuclear energy. Some experts and advocates argue that carbon-free nuclear power represents the only real hope of keeping the planet’s temperature in check. Others claim that nuclear is risky, unnecessary and far too expensive to make a dent.
The same basic data set — nuclear plants currently in existence, those under construction, the status of new technologies, the history of costs and delays, and a few striking accidents — produces those totally contradictory opinions and predictions. Nuclear power is a Rorschach test: You see what you want to see — a rosy nuclear future or an old-world dinosaur in a slow death spiral — reflecting your own views on the energy present and future. In all likelihood, no one will be proven right or wrong for decades.
Today and tomorrow
Nuclear power today accounts for around 10% of the total electricity generation around the world. This varies sharply by country — in the U.S. the rate is about 20%, in Russia and Germany it is a bit lower than that, while some other European countries get 40% and 50% from nuclear reactors. France has long led the way proportionally, at more than 75% percent. (It has the second most total reactors, behind the U.S.) China, though building rapidly, drew less than 3% of its power from nuclear in 2014.
There are 442 reactors currently in operation globally, and the International Atomic Energy Agency says 66 are currently under construction. Twenty-four of those are in China; no other country is currently building more than eight.
That’s the nuclear landscape now. The question is, how will it change in the coming years? And equally important, how should it change? The answers to both of these depend on whom you ask.
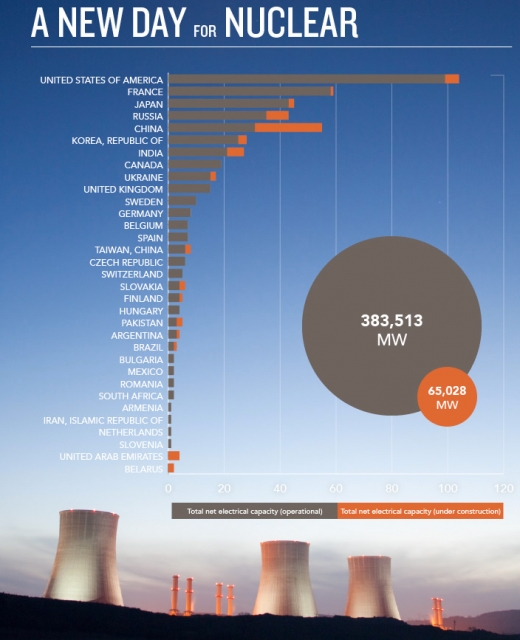
The International Energy Agency’s World Energy Outlook 2014, which includes a close analysis of nuclear power, projects a 60% leap in global installed capacity by 2040, with almost half of that growth coming from China.
“I think we definitely need it in the battle against climate change. This is broadly recognized,” says Jacopo Buongiorno, a professor of nuclear science and engineering at the Massachusetts Institute of Technology. “Because now there is such an overwhelming concern about climate change, it’s like a tide that lifts all boats. Anything that is perceived as clean is going up. I think it is absolutely necessary.”
That type of take on nuclear isn’t particularly hard to find, but neither is this one: “I don’t think nuclear power is a necessary component at all,” says M. V. Ramana, a research scholar at Princeton’s Nuclear Futures Lab. “Nuclear power as a share of electricity generation is only likely to decline in the foreseeable future. If we hold that up as a means of emission reductions, then we will not be successful with meeting any of the ambitious climate goals set” in the recent Paris agreement, in which 195 countries agreed to reduce emissions sharply.
In the run-up to that agreement, a group of the most prominent nuclear proponents — climate scientist James Hansen, Stanford’s Ken Caldeira and others — wrote in the Guardian that “nuclear will make the difference between the world missing crucial climate targets or achieving them.”
This was met with particularly harsh disdain from Naomi Oreskes, Harvard science historian and co-author of Merchants of Doubt, who wrote a response at the Guardian branding this “a new, strange form of denial.”
Will nuclear have to be part of the mix?
The heart of Hansen’s and Oreskes’ disagreement regards the necessity for nuclear and the technical feasibility of scaling up renewables: Are other energy sources sufficient to wean us from fossil fuels? Or is the reliable, large-scale (a single new reactor can reach 1,600 megawatts capacity, three times the size of the world’s largest solar plants) baseload power that nuclear provides a necessary component of the low-carbon future?
The anti-nuclear side of the argument focuses on several studies that have illustrated a renewables-only way to the goal, which could be cheaper and free of the risks associated with nuclear. Mark Jacobson, director of the Atmosphere/Energy Program at Stanford University, has published state-specific plans showing how 100-percent renewables penetration would be achievable. The National Renewable Energy Laboratory, part of the U.S. Department of Energy, published its “Renewable Electricity Futures Study” in 2012 and explained a clear path to 80 percent penetration in the U.S. Others have shown similar routes forward.
When it comes to any energy source, it is cost that sits at the root of the discussion. Nuclear proponents argue that there are impediments to having a grid entirely run on renewables. Buongiorno, for example, says that the intermittency of solar and wind can realistically only be addressed by adding large amounts of electricity storage (in the form of large batteries or other newer tech such as compressed air) to the grid, and that would change the ongoing “renewable prices are plummeting” narrative.
“When I hear people say ‘Oh, the costs are coming down,’ the costs for generation may be coming down, but if installing that capacity forces me to have energy storage, you have to add those costs,” he says. Think of it like buying a car: The baseline price sounds okay, but it’s all the options and add-ons that’ll get you. Buongiorno says he expects the costs of nuclear construction will come down, and that when storage costs for renewables are factored in, nuclear — with its reliable, 24/7 output — starts to look much more attractive as an alternative.
Costs are a key consideration
Adding more nuclear to the grid could reduce some of the burden on renewables and storage, but the economics of nuclear itself could prove an insurmountable roadblock.
In general, the more experience accumulated with a given technology, the less it costs to build. This has been dramatically illustrated with the falling costs of wind and solar power. Nuclear, however has bucked the trend, instead demonstrating a sort of “negative learning curve” over time.
According to the Union of Concerned Scientists, the actual costs of 75 of the first nuclear reactors built in the U.S. ran over initial estimates by more than 200%. More recently, costs have continued to balloon. Again according to UCS, the price tag for a new nuclear power plant jumped from between $2 billion and $4 billion in 2002 all the way $9 billion in 2008. Put another way, the price shot from below $2,000 per kilowatt in the early 2000s up to as high as $8,000 per kilowatt by 2008.
Steve Clemmer, the director of energy research and analysis at UCS, doesn’t see this trend changing. “I’m not seeing much evidence that we’ll see the types of cost reductions [proponents are] talking about. I’m very skeptical about it — great if it happens, but I’m not seeing it,” he says.
Some projects in the U.S. seem to face delays and overruns at every turn. In September 2015, a South Carolina effort to build two new reactors at an existing plant was delayed for three years. In Georgia, a January 2015 filing by plant owner Southern Co. said that its additional two reactors would jump by $700 million in cost and take an extra 18 months to build. These problems have a number of root causes, from licensing delays to simple construction errors, and no simple solution to the issue is likely to be found.
In Europe the situation is similar, with a couple of particularly egregious examples casting a pall over the industry. Construction began for a new reactor at the Finnish Olkiluoto 3 plant in 2005 but won’t finish until 2018, nine years late and more than US$5 billion over budget. A reactor in France, where nuclear is the primary source of power, is six years behind schedule and more than twice as expensive as projected.
“The history of 60 years or more of reactor building offers no evidence that costs will come down,” Ramana says. “As nuclear technology has matured costs have increased, and all the present indications are that this trend will continue.”
Cost declines are rare
Some experts, however, dispute the idea that the “negative learning curve” is intrinsic to the nuclear industry. In a recent paper Ted Nordhaus of the energy think tank The Breakthrough Institute pointed out that the history of nuclear plant construction costs varies dramatically by country. South Korea, for example, has demonstrated a fairly consistent drop in costs over time; it imported its first designs from foreign companies with more experience before homegrown designs took hold, and all the country’s plants are built and owned by a single utility. Nordhaus wrote, “with the right policies and institutions, nuclear plants can be built quickly, safely, and cheaply.”
Still, most countries have seen costs increase. As it stands, only China’s non-free market may allow for a truly rapid build-out of nuclear plants; the country’s current domination of the nuclear construction world reflects this idea, and the 2016 Five-Year Plan includes provisions to approve and build six to eight new plants each year.
The industry, for its part, argues that the benefits of nuclear are worth the price tag. The Nuclear Energy Institute, which represents plant owners, builders, designers, suppliers and related companies, notes that in the U.S. nuclear power generates as much as $50 billion each year from electricity sales and revenue, and provides around 100,000 jobs. The lack of carbon emissions, of course, only adds to the benefits.
The Fukushima shadow
Along with price hikes, the specter of major accidents hovers over every discussion of a nuclear scale-up. By most measures nuclear power is among the safest forms of energy ever devised. But when it does go wrong, it goes wrong in spectacular and terrifying fashion.
The accident at Fukushima Daiichi in Japan in 2011 led to a shutdown of all the plants in that country (with very limited reactor restarts coming only last year), and it has convinced Germany and Belgium to phase out the energy source entirely. Though those phase-outs will account for only a handful of total reactors, they put a damper on the idea of a revolutionary nuclear scale-up.
Many argue the fearful reactions and phase-outs are not entirely logical in the context of climate change. Fukushima clearly did result in a drop in global support for nuclear energy, but public opinion continues to vary sharply by country.
In the U.S., a Gallup poll on nuclear favorability has shown a decline since Fukushima, but not a dramatic one. In 2015 public support for the use of nuclear energy hovered at 51%, down from a peak of 62% in 2010. The same poll, though, found that only 35% percent think the government should place “more emphasis” on nuclear; for comparison, 79% want more focus on solar power.
Cousins to the fear of a massive meltdown are both the worry over nuclear weapons proliferation and concerns over waste disposal. Spent nuclear fuel is currently stored on the site of nuclear plants in pools of water or sealed in dry cask storage, and decades-old arguments over geologic repositories are unlikely to be resolved any time soon.
With regard to weapons, nuclear plants produce plutonium during the course of their reactions, which can be made into bombs if enough is accumulated; terrorism and theft are thus constant worries. Both of these issues work to extend the shadow of risk stretching out behind nuclear power, and both lack immediate solutions.
Technological breakthroughs?
Supporters of nuclear power hold out hope that new technologies will improve the economics and reduce the fear factor. There are ongoing efforts to develop small modular reactors, which produce about a third or less of a full-size reactor’s output and can theoretically be built faster and cheaper.
Allison Macfarlane, director of George Washington University’s Center for International Science and Technology Policy and the former chairman of the U.S. Nuclear Regulatory Commission, notes that of the various companies working on these, only one (NuScale Power) is currently expected to actually submit application materials to regulators in 2016 — a step that is still years removed from actual functioning reactors.
Other technological unicorns, though in many cases on the drawing board for decades, still remain off in the distance: different fuel sources such as thorium, molten salt-cooled reactors, even building plants on floating platforms like those used for oil drilling (a project that Buongiorno at MIT is heavily involved in) are all on the table.
These have varying potential advantages: A floating plant could use seawater as a cheap and easy way to cool the reactor and would alleviate some of the safety fears by keeping the plant away from people and near a coolant should an accident occur; thorium could reduce waste and produce power more efficiently, though a U.K. government report in 2013 called the benefits “overstated” and experts have warned it could increase proliferation risks; and molten salts can operate at lower pressures than standard water-cooled reactors, offering some safety benefit.
Nuclear research and development, though, moves at a snail’s pace, largely for safety reasons. If the goal is rapid emissions reduction, it is unclear if any of this new tech can play a role.
“I think we need to do some work on it, see if we can develop some new technologies, but they are not going to be a solution in the near term at all,” Macfarlane says about the small modular reactors. “Some of these other things that just exist on paper right now? I think they’re much further out.”
Clemmer, of UCS, agrees that the next 15 years or so are unlikely to feature much of a nuclear revolution. He says the 2030 to 2050 period, though, will be a crucial time for nuclear, with many existing plants in the U.S. and elsewhere due to retire — the IEA projects almost 200 reactor retirements by 2040. In that time frame, perhaps some of the new technology could make it to market.
Changing perspectives
In the coming years, it may come down to just how dramatic the effects of climate change become to force the Rorschach muddle to resolve into a clear image.
“As time goes on, and the impacts of climate change become more and more real — droughts and heat waves and sea-level rise and storm surge, coastal flooding issues, more powerful hurricanes and devastating storms and things like that are also a wake-up call to people,” says Clemmer. “Hopefully at some point it will be enough of a wake-up call that we’ll be demanding action to address climate change and reduce emissions. In that world, maybe there’s more of a positive light that would be shed on nuclear.”
Macfarlane also suggests that the changing perspectives on energy requirements could shift nuclear fortunes. “We go through these different transitions as a society,” she says. In the past, these transitions have replaced wood with coal to help cities grow, and added oil to feed a boom in transportation.
“Nuclear never fulfilled one of those kinds of needs,” she says. “We’re going through another transition where we need to decarbonize our energy sources, and maybe it will fill more of a natural need now. We’ll see.”
Dave Levitan is a freelance science journalist. This post originally appeared at the website Ensia.
Weekly Newsletter
Get building science and energy efficiency advice, plus special offers, in your inbox.
8 Comments
Dilithium and fusion reactors coupled with a hyper-drive are
right around the corner. IJS. ;)
Too cheap to meter, or too expensive to matter?
Given that utility market structures are currently making even EXISTING light water reactors uneconomic, it's pretty clear that the traditional big-iron doesn't (or shouldn't) have a future without subsidy. In the face of cheap natural gas and even cheaper wind power a number of midwestern nukes have either already closed or will be closing soon.
The standard industry line is the same as Buongiorno's:
"Buongiorno says he expects the costs of nuclear construction will come down, and that when storage costs for renewables are factored in, nuclear — with its reliable, 24/7 output — starts to look much more attractive as an alternative."
That "...reliable, 24/7 output..." is actually appropriately viewed as "...inflexible, 24/7 output...", in that the ramp rates on nuke are slow they can't track load or stabilize voltage or frequency, which means that beyond some minimum level they don't really work well as a primary source of power, and with even slower ramp rates than inflexible coal plants they don't "play nice" with intermittent & variable renewables OR intermittent & variable load, which is how heavilty nuclear France is stuck exporting power overnight selling power at a loss, then having to import power to support the daily peak loads, paying a premium. The intermittency issue and the amount of storage needed to address it is usually overplayed too. Over a reasonable geographical size the output of wind & solar is quite stable & predictable- more predictable than the day-ahead market load. As grids get smarter and markets are formed to remunerate demand response, loads can and are shifted in time to make up for predicted & immediate shortfalls in grid generation. The notion that stable 24/7 fixed output "baseload" power is necessary or even desirable is a myth.
Storage and demand response are useful for managing load peaks and for voltage & frequency control with or without intermittent renewables, and are arguably as much of a solution for the inflexibility of nuclear plant output as it is for the variable output of intermittent renewables, since it allows the plant to run at a higher average output, even when the total generation output is higher than the load. The amount of storage required depends on the total grid mix and how well the load is managed on the demand side.
Replacing a gigawatt nuke with renewables, demand response, efficiency and some amount of storage is not only possible, it's cheaper than a new gigawatt nuke, can be better distributed to where the power is needed, requiring LESS transmission grid infrastructure capacity. (Shall we add the transmission infrastructure cost to the napkin math of large scale nuclear, the way the cost of storage is being pushed onto the distributed renewables plate?)
Just yesterday in California Pacific Gas & Electric submitted a proposal to the California Public Utilities Commision to close the 2.3 GW Diablo Canyon nuclear plant in nine years (2025) with all non-carbon-free renewables, demand response and storage:
http://www.utilitydive.com/news/pge-to-close-diablo-canyon-nuclear-plant-replace-it-with-renewables-effi/421297/
It takes longer than nine years to just get the permitting and financing in place, let alone actually build a 2.3 GW nuclear plant!
If there is a future for nuclear in the US (and I think there is), it will be with walk-away-safe liquid fuel/molten salt reactors operating at atmospheric pressure (no containment structure necessary) that uses the existing stockpile of spent fuel rod from legacy reactors as fuel. The existing nuclear fleet in the US uses less than 5% of the source fuel energy before the fuel rods are too brittle to be safe, and the hazard with spend fuel rods dissipates over 10s of thousands of years. A molten salt reactor should be able to extract more than 90% of the remaining energy in that already mined & processed fuel, and the resulting spent fuel would remain a hazard for only a few hundreds of years. Even if the electricity being generated by these reactors turns out to be more expensive than renewables (which is pretty much a given), it will still be cheaper power than current reactor designs, and it will take a long step toward solving the high level nuclear waste problem.
But developing those reactors into commercial product and deploying them at scale simply can't happen in a time scale fast enough to replace all the inflexible coal fire generation in time to keep the 2C global warming targets- it's not the solution to global warming, but it is a solution to the spent fuel rod problem. In the near term there is a rationale for subsidizing SOME (but not all) of the uneconomic nuclear fleet to avoid having them pushed out of the market by expansion of cheap natural gas, but there is no need to keep them going forever.
Many of the 3rd & 4th generation nuclear plants currently under construction are destined to become stranded assets, too expensive to operate as cheap renewables and cheap storage gain further penetration into the market. Unlike big-iron nuclear, the "learning curve" of renewables and storage is steep and in the right direction, and unlike nukes, solar and storage can be scaled and co-located with the load, which reduces the ultimate capacity requirements of the grid, and makes the grid more reliable.
Watts Bar 2 took 43 years to complete, and operated for three days while ramping up to ~20% before it went into automatically shutdown due to problems earlier this month. It's fixable, but it's not at all clear that it was a wise choice to finish it. (If that's the picture of success, failure is beyond imagination!) The anticipated lifetime levelized cost of power from the over schedule and over budget Vogtle plants in Georgia are going to be dramatically more expensive than un-subsidized rooftop solar by the time they actually fire them up. These plants are the solution to anything other than the bottom lines of the utilities that own them, and get a guaranteed ROI on the capital expenditure, and when they have to retire before their full lifecycle due to competition from cheap renewables & storage they will be be albatrosses around the necks of soon to be bankrupt utilities.
Nuclear Problems
With climate change being the main point of discussion in this article, it is helpful to remember that there have been many times in the past few years that nuclear plants have had to reduce their output exactly when they have been needed most, during summer heat waves. The water temperature in their cooling ponds exceeded their design limits and could not provide the reactors with proper cooling. Large scale wind and solar actually helped balance the grids loads during these times.
That is in addition to the fact that Wall Street investors wants nothing to do with them economically.
Furthermore, the fact that there are about 150 plants that have been shutdown, seven of which were catastrophic failures (four at Fukushima, two at Chernobyl and one at Three Mile Island) means to date there is a 4.7% chance for a disastrous ending for a reactor.
Two very good videos from Rocky Mountain Institute on renewables versus nuclear and other traditional baseload power.
https://www.youtube.com/watch?v=MsgrahFln0s
https://www.youtube.com/watch?v=k3kve0PV0VA
Heat waves and droughts are problems for most thermal plants.
The water footprint of all thermal plants (any fuel) is pretty high.
France has to throttle back nukes during heat waves to avoid overheating the rivers. It's not a design limit problem for the reactor, but an environmental damage issue for the river ecosystem
India regularly has to throttle back coal fired generation during the dry seasons due to lack of cooling water, sometimes to retain sufficient water resources for agriculture & urban use. No matter how many coal plants they build in India the cooling water problem presents a limitation to the rate they can burn coal for power. The same limitations apply to nuclear plants in India, despite the active nuclear industry there clamoring for more.
In the US even nuclear plants using ocean water for cooling sometimes have to cut power to comply with regulation around localized warming of the water after weeks of hotter summer temperatures. (Again, usually for environmental reason rather than design limits on the powerplant.)
PV solar output also takes a hit at high ambient temperatures, but it doesn't drop to zero, and it doesn't use water.
Using the dumb-math odds based on history of different reactor designs and site locations to calculate the risk of catastrophic failure is just that- "dumb". Site conditions and associated risks vary widely, some are known, others not. Very few sites have Fukushima-like tsumami susceptibility (zero, now that the example has shown the weakness of siting the backup gensets), and almost no powerplants outside of the old Soviet bloc have the same lack of containment seen at Chernobyl. Safety systems have been incrementally improving since the TMI incident. Calculating the odds of a catastrophic failure at any one powerplant or even across a large fleet takes a much more sophisticated approach than simply counting the number of closed plants and the number that closed due to disasters. The scale and impact of the disasters vary considerably too. TMI barely counts, compared to what happened at Chernobyl or Fukushima, given the differences in impact on the local and larger regions. That said, the seismic risks to Diablo Canyon is reason enough to retire it early, taking an abundance of caution.
The most common end of operation for reactors are economic, not physical disaster, and that will continue to be the case (at an accelerating rate.) The current US fleet is pretty old, and many need expensive refitting to keep running. Sometimes that works reasonably economically, sometimes it doesn't (most spectacularly in the case of the San Onofre Nuclear Generation Station that they had to give up on). Upgrading an old nuke going is still a lot cheaper than building a new light water reactor, and can usually be done in much less time, but re-fitting an old nuke can be more expensive than new renewables.
The reasons Wall Street won't get into the game isn't at all about physical failure risk- it's all about the economics and the time/money scales being to large, colored by the catastrophic 1982 bond failure of the Washington Public Power Supply System build out of large numbers of plants (way over budget and way off schedule) that had no apparent market for the output to begin with. That was before Chernobyl, before Fukushima, and it pretty much ended private sector financing of nuclear projects in the US.
Catastrophic failures of molten salt reactors are essentially impossible, but since there has yet to be a commercial MSR powerplant built, the cost is still really unknown. There is active private money being invested in small fail-safe nuclear technology and some of those designs will find a market, just not in sufficient time to make much of a difference in the climate change problem. By comparison PV and wind are truly mature technologies, but still emerging upstart adolescents in the overall energy biz. It's doubtful that MSRs and other small nukes will be able to cost-reduce and scale fast enough to push out wind & solar. We'll see (maybe by 2030, but not sooner.)
The Future of Nuclear
You might look at this article by Amory Lovins.
http://www.forbes.com/sites/amorylovins/2016/06/22/close-a-nuclear-plant-save-money-and-carbon-improve-the-grid-says-pge
@Dana, It's unfortunate that the US developed MSR
tech back in the early 1960's but the industry opted against its and I never understood why.
I agree completely with Dana, "but wait, there's more!"
International Energy Agency forecasts have been consistently low for renewables: can we trust them for nuclear? I would not be surprised if nuclear shrinks in the next couple decades, although I'd be delighted if cost, speed, and safety issues are solved and it booms.
(sorry, that was unintentional).
Nuclear advocates say that it provides over 100k US jobs? Well, PV alone already provides over 200k (http://www.thesolarfoundation.org/national/)... and I wonder if that 100k includes the multiplier effect?
Thanks for the article: seems accurate and fair.
The IEA isn't any better than the EIA on predicting this stuff.
The IEA predicts continuing expansion of oil use too, but fails to elucidate where that much oil can possibly be sourced at the predicted levels. The IEA is only slightly better then the EIA at predicting the past too.
There really isn't much of a chance of MSR technology "booming" even under the artifices of electricity market designs, but the added-value of processing the spent fuel rod into something more manageable makes it more likely to gain traction as a subsidized bit-player in electricity markets. Most of the spent fuel rods are located where large scale grid infrastructure already exists, on real estate already designated as a nuclear power plant site, which should make getting the permitting easier and the overall costs lower, and the waste would not have to be secured & transported to remote sites.
The electricity output is just the (fairly substantial) gravy on top of the waste reduction meal. Mining thorium for fuel and building on new sites makes almost no sense for most of the nuclear-developed world. The total energy delivered by MSRs using spent fuel rod will be more than 15x the total amount of power that came out of the original reactors that used those fuel rods.
That's a LOT of total lifecycle electricity to be harvested from the existing hazardous waste problem- enough to power the entire world at current electricity use levels for 50 years or more. That's not to say there is a strong need to burn through that existing stockpile at anywhere near that pace though, especially when it's more expensive than renewables + storage (and it likely will be by the time the technology is in commercial production.) It can be considered a waste processing technology that mostly pays for itself. How fast the waste gets processed will depend on other political & economic factors, not the electricity markets.
The reasons why MSR technologies weren't developed into commercial power in the past 50 years has many layers, much of it political and geopolitical during the cold war arms race. It's a whole lot harder (but not impossible) to refine weapons-grade isotopes from MSR fuel waste than from spent fuel from other reactor types, and building on improving those other designs was deemed to be the financially safer path once the industry had already been established. In some of the developing world there may be a case for mining thorium &/or uranium for fueling MSRs as a replacement for existing coal plants, but the economic case still seems pretty weak in the face of rapidly declining renewables & storage costs. MSRs are more scalable than LWRs, but still require greater grid infrastructure than load-sited PV + storage.
From the 1960s through the 1980s (almost) nobody was predicting that demand growth would slow down (largely due to efficiency gains, much of it by government mandated efficiency minimums, not market forces), or that the inflation adjusted price of electricity would keep declining. By the 1990s many were getting an inkling, but even then nobody was predicting the flat-to-falling demand of the past decade. In the past year for the first time more non-hydro renewables were deployed in the world than the net increase in worldwide demand(!), and that is likely to accelerate as the technology improves and costs fall. In 2016 something like 2/3 of the planned new capacity in the US will be non-hydro renewables, also a first. (Combined cycle gas had been the 800 lb, gorilla to beat in recent years.) New MSRs would have a real problem surfing the renewables tsunami even if they were already commercialized. Catching up as a "me too" low-carb technology is going to be difficult- probably impossible.
For a peek at the inflation adjusted price of electricity over time, the EIA's price viewer is as good as any. Select "Electricity" from the pull down tab, and scroll back to 1959. In 2015 dollars the US average price of electricity in 1959 was 21 cents rather than the current 12-13 cents. The current average price has been flat (inflation adjusted) for about 20 years, but with downward pressure from cheap renewables it's probably going to take a turn down over the course of the 2020s.
http://www.eia.gov/forecasts/steo/realprices/
Per-capita and total electricity use in the US was still on an upward trend until about 2005, after which it's been pretty flat or falling since:
http://www.statista.com/statistics/201794/us-electricity-consumption-since-1975/
With more behind the meter efficiency, storage, and PV, those numbers are ripe for further reducing the size of the market any grid source will be able to sell, into (including large scale wind/solar and of course, MSRs.) Even with rapid electrification of the transportation sector, total US grid demand isn't likely to grow much (if at all) due to proliferation of behind the meter resources.
The conditions for an actual boom in MSR deployment doesn't really exist in the US, but maybe in China (?). India may have room for boomlet due to increasing demand, but will be cooling water resource limited. A sustained boom would require MSR power to be so cheap that retiring the existing coal fleet early becomes economically attractive, but there are also political obstacles to overcome to make that happen given the scale and influence of the state owned Coal India Ltd near-monopoly.
Bottom line, MSRs are really kewl and all, but the market isn't likely to be all that huge by the time the technology is ready for wide commercial deployment. If they ever comprise a double-digit percentage of the world's electricity supply I'll be surprised. (Nuclear has a very low double-digits share worldwide, maybe 20% of the US market right now.) PV alone will hit a double-digit fraction by 2020, 2022 at the latest (up from less than 2% presently), and probably a quarter of the pie by 2035. In that time frame the costs of renewables will have dropped to the point that nuclear (even MSR) will be too expensive to even matter, except for the waste processing value.
Log in or create an account to post a comment.
Sign up Log in