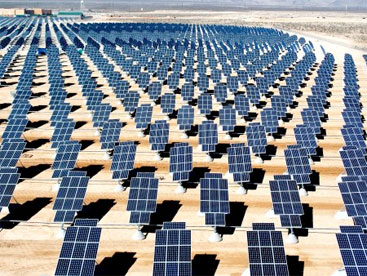
Almost all of our methods for generating electricity are fundamentally the same. Coal-, nuclear-, and natural-gas-fired power plants boil water to produce high-pressure steam that spins a dynamo in a “steam turbine.” The wood-chip-fired combined-heat-and-power (CHP) plant that we want to build in Brattleboro works the same way. The heat sources used in these systems, of course, are very different—each with its own environmental and health concerns—but the way they actually produce the electricity is, fundamentally, the same. Hydro power plants use the gravitational force of flowing water to spin a similar turbine, and windmills use the power of wind to do the same thing.
There’s only one fundamentally different power-generation technology that’s feeding a significant amount of electricity into the utility grid: photovoltaics, or the direct conversion of sunlight into electricity. Photovoltaic (PV) cells, remarkably, produce electricity with no moving parts. There’s no mechanical gearing to lubricate or wear out, essentially nothing to maintain. They just sit there, in the sun, and generate direct-current electrical energy.
While simple in their effect, how PV cells work is quite complex. Here’s an oversimplification of the process: A PV cell is made of a “semiconductor” material, such as silicon—semiconductors are materials whose electrical conductivity can be changed by adding small amounts of impurities. In a typical silicon PV cell, there are two layers of slightly different silicon, separated by a “cell junction.” On one side of the cell, the silicon wants to get rid of electrons; the other side wants electrons.
When photons of light strike the cell, the electrons in the silicon become energized, and some of them jump across the cell junction—but they con only cross that junction one way. As a result a charge differential is created, with more electrons (electrical charge) on one side of the cell than the other. By connecting a wire to the cell, this charge differential can be equalized by allowing the electrons to flow through the wire. This flow of electrons is direct-current (DC) electricity, and we can tap into that current to perform work—powering motors, producing light, etc.
PV cells are wired together into “PV modules” so that the small amounts of current created by each cell are aggregated into useful quantities of electrical power. The modules, in turn, are often combined into “PV arrays”—sometimes huge arrays that generate many kilowatts or even megawatts of electricity when the sun is shining.
Finally, inverters convert the DC electricity into alternating current (AC) that can operate standard electrical devices or be fed into the power grid using special equipment.
The practical photovoltaic cell was invented in the 1950s by scientists at Bell Laboratories in New York. Initially, the cost was extremely high—thousands of dollars per peak watt of output. The first applications for PV were on space satellites where that high cost was not a concern. In fact, local PV expert Richard Gottlieb of Sunnyside Solar in Guilford, VT, worked at the Naval Research Laboratory installing PV cells on the Vanguard satellite as a college student in 1957.
As the manufacturing of PV modules was streamlined and the cell efficiencies improved, costs came down. By the 1970s, PV power became practical for applications like remote radio repeater stations and Coast Guard signal buoys. In 1983, I organized a conference for the Northeast Sustainable Energy Association, “Photovoltaics: From Research to Reality,” which was held at MIT in Cambridge. By then, some pioneering designers were incorporating PV arrays into houses. Steven Strong, of Solar Design Associates in Harvard, MA, designed some of the first such homes, and he continues to be a leading designer of PV-powered homes.
Today, there are tens of thousands of homes throughout the country that derive a significant portion—sometimes all—of their electricity from PV arrays. Many of these are “off-grid” homes that are totally independent of utility power. They have battery banks so that lighting and other electrical devices can be used at night or during very cloudy periods. There are dozens of such homes in the Brattleboro area.
Other homes with PV power are “grid-connected.” Rather than batteries, these homes have “net-metering” systems that feed electricity into the grid when the sun is shining and the PV system is producing more electricity than the house needs to operate. In states with net-metering laws, including Vermont, New Hampshire, New York, and Massachusetts, when the PV system is producing excess power the electric meter spins in reverse, and the utility company effectively pays you for that electricity at the same retail price you pay for electricity.
Though PV costs have dropped a great deal since the 1980s, high first-cost remains an impediment to widespread use of PVs. For a grid-connected PV system (without batteries), installed costs today range from About $6,000 to $12,000 per peak kilowatt (kW) of capacity, before government incentives or tax credits. Thus, a 4.5 kW residential system, which would produce about as much electricity annually as a typical home uses, will cost in the range of $27,000 to over $50,000. Grid-connected PV systems are eligible for cash subsidies from the states of Vermont, New Hampshire, Massachusetts, Connecticut, and New York, as well as a 30% federal tax credit.
For more information on costs and subsidies, contact an installer, such as Richard Gottlieb or one of the companies listed in last week’s column.
Weekly Newsletter
Get building science and energy efficiency advice, plus special offers, in your inbox.
0 Comments
Log in or create an account to post a comment.
Sign up Log in