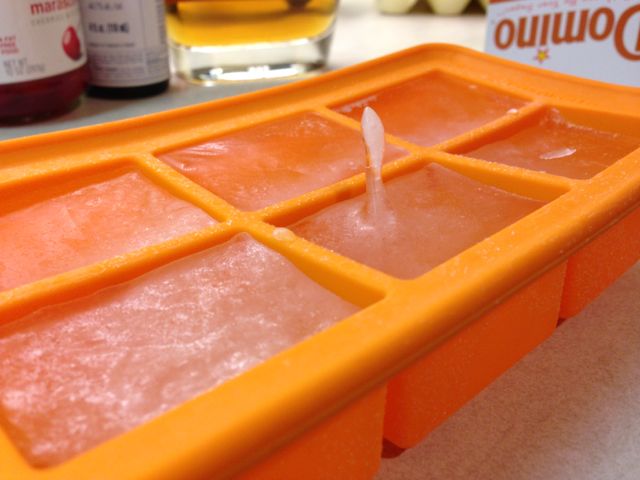
Building science is an odd subject. Few colleges and universities teach it. The majority of those who work on buildings call themselves engineers, architects, and contractors, not building scientists. And many of those who do invoke the term can explain at least one implication of the second law of thermodynamics (we’ll get to that below) but may not know what the other laws of thermodynamics are, why their numbering is so peculiar, or even how many there are. Do you?
Well, today let’s address this deficiency. If you’re going to speak of one of the laws of thermodynamics, it’s your duty at least to know the others well enough to tell a curious person what they are and which ones have some bearing in the world of building science. Before we launch into this subject, though, let’s get a little perspective on thermodynamics from the physicist Arnold Sommerfeld:
Thermodynamics is a funny subject. The first time you go through it, you don’t understand it at all. The second time you go through it, you think you understand it, except for one or two points. The third time you go through it, you know you don’t understand it, but by that time you are so used to the subject, it doesn’t bother you anymore.
With that, let’s begin at the beginning, sort of. The first of the laws of thermodynamics is not the first law of thermodynamics. (I told you it was peculiar!) It is…
The zeroth law of thermodynamics
Let’s start with the obvious question: Why isn’t it called the first law of thermodynamics? As it happened, this law was discovered after the first and second laws but considered to be more fundamental. So we have a zeroth law.
Now, the statement of the zeroth law:
If two systems each are in thermal equilibrium with a third, they are in thermal equilibrium with each other.
And that, of course, raises the question of the definition of thermal equilibrium. Let’s start with a glass of lukewarm water. If you drop an ice cube into it, is the ice in thermal equilibrium with the water? No. It absorbs heat from the water.
But if we think about that ice cube sitting in the freezer before we pulled it out. The ice is surrounded by cold air in the freezer. Once the ice has been in the freezer long enough to freeze completely and then reach the same temperature as the air in the freezer, no heat transfer happens between the ice cube and the freezer air. Now we have a case of thermal equilibrium.
Thus, two systems that are in thermal equilibrium are at the same temperature, are in thermal contact with each other, and have no net heat flow from one to the other. One consequence of the zeroth law is that temperature measurements are kind of a big deal.
The first law of thermodynamics
Depending on how you’ve come to the field of building science, you may have encountered this law. If you studied physics or engineering, you’ve no doubt seen all four laws of thermodynamics. If you instead arrived by a different route, this one may be new to you.
In thermodynamics, the first law is stated as a relation among heat, work, and internal energy. Let’s forgo that formality and technical detail here and cut to the fundamental principle that’s important to building science: Energy can be neither created nor destroyed.
Yep. The first law of thermodynamics is a formulation of the law of conservation of energy. It has many applications in building science.
Let’s begin with how we heat buildings. If it’s with a gas furnace, the fuel has a certain amount of chemical energy. When the gas gets to the burner and ignites, it changes form. The chemical reaction creates heat, which raises the temperature of the exhaust gases. Those exhaust gases travel through the heat exchanger, increasing the temperature of that hunk of metal. The blower then blows air over the heat exchanger, extracting heat from the metal and then sending it through the ducts.
Every bit of chemical energy converted to heat ends up somewhere. Ideally, you want as much of that heat as possible to end up in your house, but not all of it does. Some goes up the flue with the exhaust gases. Better heat exchangers capture more of the heat for use in the house, but all furnaces send at least some heat up the flue. Another way you lose heat is when your ducts go through unconditioned space. The bottom line, though, is that if you burn 100 units of chemical energy, you get 100 units of heat.
Here’s another way the first law works in buildings. Consider a ceiling fan. In summer, you turn it on to create a nice breeze blowing over your skin to cool you off. What happens with the energy is that you’re actually heating up the room. In this case, the incoming energy is electrical. The fan motor converts it to mechanical energy, the moving fan blades. But it doesn’t do convert electricity to motion at 100% efficiency. The wires in the fan motor have resistance, which creates some heat. Thus, some of the electricity is turned into heat right away. The rest of the electrical energy becomes mechanical energy, but even that eventually becomes heat. So all of the electricity you use to run the ceiling fan actually heats up the room, which is why it does no good to leave them on when no one is there to feel the breeze.
In his book, Buildings Don’t Lie, Henry Gifford advises readers on how to use the first law of thermodynamics to understand energy saving features and to sniff out scams. For a product to save energy, he says, you have to know where the energy is going before using that product. Insulation, for example, reduces the amount of heat lost from a building in winter, with a corresponding reduction in the amount of heat you have to add to the building to maintain comfort. If you can’t identify clearly the path of energy conversions, Gifford says, “the proposed energy-saving measure should be viewed with skepticism.”
Take Amish fireplaces, for example. Although they’re marketed as great energy savers, there’s nothing about them that saves energy over any other electric resistance heater. They all convert electricity to heat at 100% efficiency (like a ceiling fan). The only way to save energy with a space heater is if you turn off the heat in the rest of the house.
The second law of thermodynamics
Once scientists figured out the first law of thermodynamics, they began to see that it would allow all manner of bizarre things to happen. Let’s go back to our glass of lukewarm water. It would not violate the first law for the water in the bottom half of the glass to get cold, possibly even freezing into a block of ice. For that to happen, the water in the top part of the glass would have to get hot, maintaining the same amount of thermal energy in the glass.
Ever seen that happen? No. It may not violate the first law, but we know from our own direct experience that things like that just don’t happen. That’s where the second law of thermodynamics comes in. This one is a bit more complex and has several, equivalent, formulations. We could geek out over heat engines, Carnot efficiency, entropy, and the arrow of time, but for the purposes of building science, the statement of the second law by Rudolf Clausius is the one of most significance: Heat can never pass from a colder to a warmer body without some other change, connected therewith, occurring at the same time.
In winter, the heat from our fireplace naturally wants to move to the outdoors. Heat in the outdoor air doesn’t make its way into our house “without some other change.” We can get heat from the outdoor air to heat our home with a heat pump, but that piece of equipment has other stuff going on and doesn’t violate the second law. But even then, when you look at how it really works, heat still flows from the warmer to the cooler object. (For more details, see my article on how a heat pump gets heat from cold.)
Heat moving from hot to cold is the implication of the second law of thermodynamics I alluded to in the first paragraph. It’s taught in many introductory building science classes, as well as extrapolations from the flow of heat to the flow of moisture (wet to dry) and air (high pressure to low pressure).
Going just a wee bit beyond building science, the second law of thermodynamics implies that the ultimate fate of the universe is heat death. Given sufficient time, all the energy of the universe will end up spread out uniformly, with everything at the same temperature. That assumes the universe is an isolated system, of course. If our universe is simply a speck of matter in some larger universe, our fate may depend on what happens there, much as an ant’s fate might depend on which way that kid points the magnifying glass.
The third law of thermodynamics
This one’s pretty simple:
The absolute zero of temperature is unattainable.
This law is a direct consequence of the second law. One formulation says that heat engines can never be operated at 100% efficiency, with an equation defining the maximum efficiency as the Carnot efficiency:
e = 1 – TC / TH
TC and TH are the temperatures of the cold and hot reservoirs, respectively. If the cold reservoir were at a temperature of absolute zero, the efficiency would be 100%. But the second law makes it pretty clear that can’t happen. Voilà ! We need another law of thermodynamics!
Building science and thermodynamics
Building science is concerned with heat flow through building enclosures and heat supplied to or removed from conditioned space. The laws of thermodynamics lay down the fundamental rules for understanding heat, and that means if you want to understand building science better, you need to know some thermodynamics. When you get far enough into it that you know you don’t understand it but are used to it, then you’ve made serious progress in your education!
_______________________________________________________________________
Allison A. Bailes III, PhD is a speaker, writer, building science consultant, and the founder of Energy Vanguard in Decatur, Georgia. He has a doctorate in physics and is the author of a bestselling book on building science. He also writes the Energy Vanguard Blog. For more updates, you can subscribe to the Energy Vanguard newsletter and follow him on LinkedIn.
Weekly Newsletter
Get building science and energy efficiency advice, plus special offers, in your inbox.
One Comment
Thermodynamics humor
Ever since I read poet Allen Ginsberg's version, I've enjoyed funny versions of the Second Law. C.P. Snow has been credited with this very similar version. I'm not sure which came first.
Zeroth: You must play the game.
First: You can't win.
Second: You can't break even.
Third: You can't quit the game.
FFI:
https://en.wikiquote.org/wiki/Thermodynamics#Humorous
http://www.eoht.info/page/Thermodynamics+humor
https://en.wikipedia.org/wiki/Ginsberg%27s_theorem
Log in or create an account to post a comment.
Sign up Log in